X-ray Crystallography
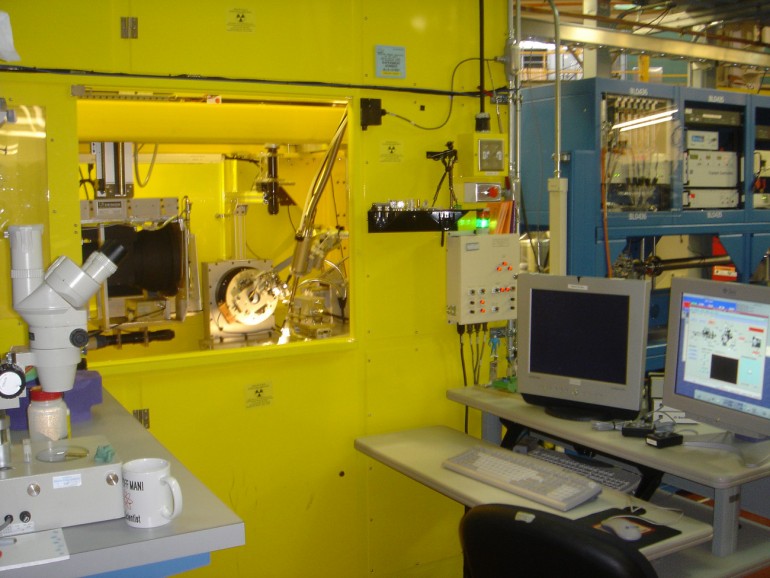
The 4.2.2 beamline hutch at ALS Berkeley
McGovern Medical School is a founding member of the Molecular Biology Consortium (MBC), which constructed and currently operates MAD-capable X-ray beamline 4.2.2 at the Advanced Light Source (ALS) synchrotron (Berkeley CA): a superconducting dipole magnet source (a “Superbend”).
By virtue of our part ownership of this beamline and payment of an annual maintenance fee, we at McGovern Medical School have access to the beamline and MBC staff, including scheduling personal trips to the beamline at Berkeley for hands-on use with operator assistance. Furthermore, in 2007 we set up the capability for remote control of the beamline by computer access from workstations in our laboratories in Houston. The beamline uses Citrix client/server software, permitting remote, password-protected online access to the beamline controls (often called “FedEx crystallography”). Crystals are shipped to beamline staff who load the crystals into a Rigaku robot.
The Rigaku robot allows users to remotely access the beamline, control crystal positioning, define protocols for X-ray exposure for screening or full diffraction data collection, and data transfer to the workstation in the users home institution. This remote control capability has been implemented by the Center for Membrane Biology of the Department of Biochemistry and Molecular Biology. (Note: If a remote user needs help after hours, they can call the support person, who can examine the problem from home, over the internet. Usually this process is sufficient to solve problems. Some problems require physical intervention by the support person, and all three support personnel are within 30 minutes driving time from the beamline. Our experience is that users are well supported by this process.)
Technically, beamline 4.2.2 is almost ideal as a macromolecular crystallography station. Its 5T dipole magnet source, in the 1.9 GeV ALS electron storage ring, provides a spectrum with a critical energy of 12 keV, ideal for X-ray diffraction of macromolecule crystals. The source is small and its angular width is also small, so it is possible to achieve a very tight focus of the X-ray beam with little angular divergence, with good X-ray optics. The X-ray optics of 4.2.2 were designed by Dr. Gerd Rosenbaum, and implemented by L.R. Design of Scottsdale, AZ (Larry Rock). These are essentially the same optics as have been implemented at many APS and NSLS beamlines, among which is APS beamline 19-ID, the world’s most productive structural biology beamline. ALS 4.2.2 is the first West Coast beamline utilizing Rosenbaum-Rock optics. Beamline 4.2.2 provides high flux and high flux density over a wide spectrum: 5 – 15 keV. Over this energy range, beamline 4.2.2 delivers a total flux exceeding 1012/s into a focused spot 50µm vertical, and 125µm horizontal (flux density exceeding 1.5 x 1014/s/mm2). The beam is essentially parallel, with a Gaussian cross-section. The energy resolution is that of its Si(111) monochromator crystals: about 0.00014, or about 1.7 eV at the selenium edge (12.66keV). These measured parameters indicate that ALS 4.2.2 is among the top beamlines in the world in terms of technical performance.
NOIR Detector
One unique feature of the NOIR detector system is that the light from its phosphor converting film is coupled to the CCD by a lens, rather than a fiber-optic taper, used in all other commercial protein crystallography detectors. The lens was developed by Optics One, Inc. of Westlake, CA, through a contract with the MBC, during 2002-2003, with financial support from NIH grant R01 RR16334 (Edwin Westbrook, P.I.). This lens exhibits essentially no geometric distortion: in mapping its image onto a Cartesian system, no point on the image field deviates more than 0.2 pixels (16µm) from its place in Cartesian coordinates. Data recorded on a NOIR detector can be processed without geometric distortion correction, with almost no discernable data quality degradation. Also, in contrast to the situation with a fiber-optic taper, the lens response sensitivity is smooth. Fiber-optic tapers exhibit abrupt discontinuities, called “shear distortion” where neighboring fibers lose contact with each other. Also, fiber-optic tapers exhibit abrupt changes in sensitivity between neighboring pixels, of up to 50% in many cases. “Chicken-wire” nonuniformity is due to imperfections at boundaries between fiber-optic bundles in the tapers, which are made by pressing bundles together to make the large fiber cylinders, prior to pulling them into taper shapes. The larger the fiber-optic taper, the more imperfections it contains. Although spatial nonuniformity can be corrected, to first order, with appropriate flood-field images (Pflugrath, 1997), this processing introduces subtle, undesirable errors (Thomas, 1990). Smooth uniformity correction, as manifest by a lens, avoids these problems.
Another problem of fiber-optic tapers, that the lens avoids, is light scatter within the taper. The concept that a glass fiber completely contains transferred light, is true only if the fiber is optically isolated. Light however does couple between adjacent fibers if they touch. To reduce this effect, fiber-optic manufacturers introduce black fibers into the bundles: typically one in six fibers is black. This “extramural absorber” (EMA) reduces transparency by ~20%, but it does sharpen the image by reducing light scatter. However, some scatter remains, and it is a well-known feature of fiber-optics that their images exhibit “tails” that extend quite far from a light stimulus, at low levels (0.1% – 1% of the peak value, up to 750µm from a point source). Such tailing is not seen in lens optics, which provide an essentially perfect Gaussian response.
The NOIR system uses a thin (~50µm) Gd2O2S:Eu phosphor film to convert incident X-rays to visible light. The output spectrum of Gd2O2S:Eu has several emission lines spreading from 580nm – 720nm. Therefore, the lens that is used in the NOIR system has been designed to focus this entire spectrum: the lens is “achromatic.” The MBC specified that the Optics One lens focus 99% of all light in this spectrum, from a point source, within a circle of radius 20µm. The MBC has demonstrated that the lens meets this specification. Of course, the phosphor degrades the point response of the lens, because the phosphor scatters light generated within it. But the measured point response of the NOIR detector is sharper and tighter, than any fiber-optically coupled CCD system tested to date, including the ADSC, MAR, and ESRF “Frelon” systems (John Morse, personal communication).