van Hoof Lab
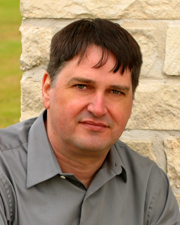
Ambro van Hoof, Ph.D.
All life forms must maintain a homeostatic gene expression program, which is achieved at many different steps in gene expression. The generation of mature functional RNAs requires a wide variety of RNA processing steps that are each tightly regulated to control gene expression. Many of these RNA processing reactions require RNases. RNases are also critical because they degrade RNAs when they become damaged, are misprocessed, or are no longer needed. Thus, during its life-time each RNA molecule is acted on by a number of different RNases. The importance of RNA processing and degradation extends across all RNAs, including mRNAs and a variety of non-coding RNAs. While some of the RNases involved are highly specialized in one function, others are very general. For example, the RNA exosome carries out both processing and degradation reactions in both the nucleus and the cytoplasm and its substrates include both mRNAs and ncRNAs. Every eukaryotic genome encodes dozens of RNases. Many of these RNases are mutated in human disease, including cancers and human mendelian syndromes, but we don’t understand what mRNA or ncRNA these RNases digest and whether they function in RNA maturation or degradation. For example, pontocerebellar hypoplasia is caused by single amino acid changes in either the RNA exosome or the tRNA Splicing EndoNuclease (TSEN). We do know some of the functions of these multisubunit enzymes, but not all of the functions. We don’t know what functions are relevant to the disease, or the mechanism by which single amino acid changes affect these functions.
The van Hoof lab studies how RNases contribute to the eukaryotic gene expression program, using the yeast Saccharomyces cerevisiae as a model organism. The van Hoof lab studies many different RNases, but one focus of our attention has been how the RNA exosome acts on a wide variety of RNAs, yet is very specific for those RNAs. For example it degrades normal cellular mRNAs very slowly, but degrades aberrant mRNAs very rapidly. These aberrant mRNAs include mRNAs that have been cleaved by RNAi or any other RNase, mRNAs that lack a stop codon and viral mRNAs. We take advantage of the known structure of the RNA exosome and the power of yeast genetics to understand the mechanisms by which the RNA exosome acts specifically on its substrate RNAs.
One explanation for why TSEN and RNA exosome mutations both cause pontocerebellar hypoplasia is that they may act in concert to degrade some specific RNA during neuronal development. It is therefore important to understand TSEN specificity. TSEN derives its name from its ability to cut introns out of tRNAs, and has been studied for that role for decades. We used yeast genetics combined with transcriptome sequencing to identify a small number of other mRNAs cleaved by TSEN, and map the cleavage sites, thereby expanding the known role of this RNase.
These and other projects on endoribonucleases, 5’ and 3’ exoribonucleases and decapping enzymes are described in our recent publications. For lab news, check @vanhooligans on twitter.